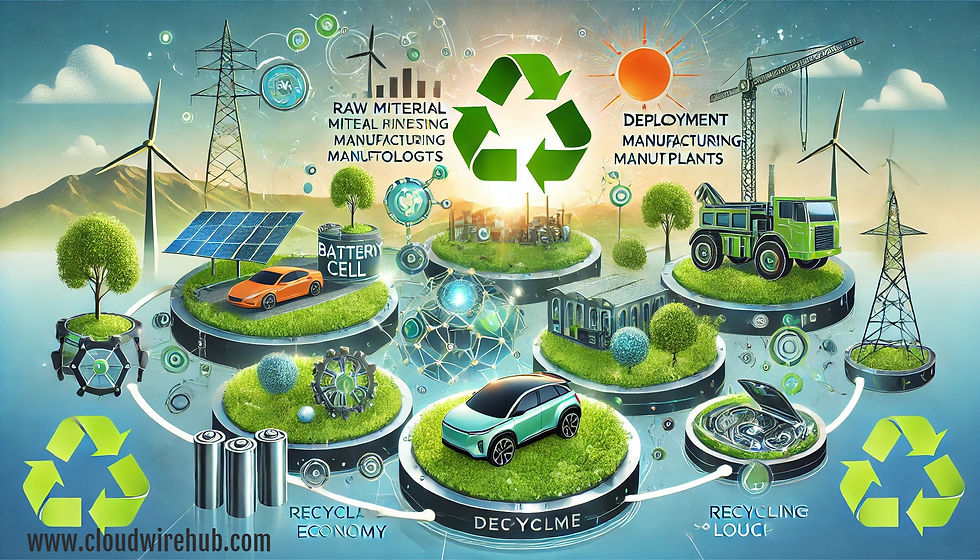
Introduction
In today’s world, batteries are the linchpin of modern innovation, driving technological advancements across industries. From electric vehicles (EVs) to renewable energy storage, the battery has become a cornerstone of sustainability and energy transformation. However, behind every battery lies a complex and interconnected value chain—a sequence of activities that takes raw materials from the earth, processes them into usable components, and delivers the final product to the consumer.
This article explores the battery value chain, from resource extraction to recycling, highlighting the opportunities, challenges, and innovations shaping its future.
What is the Battery Value Chain?
The battery value chain encompasses all processes involved in creating batteries, from raw material sourcing to end-of-life recycling. It is divided into several stages:
Raw Material Extraction: Mining key resources such as lithium, cobalt, nickel, and graphite.
Material Processing: Refining raw materials into battery-grade compounds.
Cell Manufacturing: Producing the cells that make up battery packs.
Battery Assembly: Integrating cells into modules and systems.
Deployment and Use: Batteries in products such as EVs, electronics, and energy grids.
Recycling and Reuse: Recovering materials to minimize waste and environmental impact.
Each stage is interdependent, and improvements in one link can enhance the overall chain’s efficiency and sustainability.
Key Components of the Battery Value Chain
1. Raw Material Extraction
The foundation of any battery lies in its materials. Lithium-ion batteries, the most common type, require critical elements:
Lithium: Found in brine pools and hard rock deposits, primarily in Australia, Chile, and Argentina.
Cobalt: Mined mainly in the Democratic Republic of Congo (DRC).
Nickel: Sourced from mines in Indonesia, the Philippines, and Russia.
Graphite: Extracted from China, Mozambique, and Canada.
Challenges in Raw Material Extraction:
Environmental Impact: Mining can devastate ecosystems, consume large amounts of water, and generate significant emissions.
Ethical Concerns: Artisanal mining, especially for cobalt in the DRC, is fraught with child labor and unsafe working conditions.
Efforts to address these challenges include adopting sustainable mining practices and diversifying supply chains to reduce dependence on specific regions.
2. Material Processing
Raw materials must undergo extensive processing to meet the stringent purity requirements of battery manufacturing. For example, lithium is transformed into lithium carbonate or lithium hydroxide, while cobalt is refined into battery-grade cobalt sulfate.
Key Considerations in Processing:
Energy Intensity: Processing often requires large energy inputs, contributing to carbon emissions.
Geographic Concentration: A significant portion of material processing occurs in China, creating vulnerabilities in global supply chains.
To reduce emissions and increase resilience, companies are exploring innovations such as direct lithium extraction (DLE) and localizing processing facilities.
3. Cell Manufacturing
Battery cells are the building blocks of battery packs. This stage involves combining processed materials to create cathodes, anodes, electrolytes, and separators, which are assembled into cells.
Technological Innovations:
Solid-State Batteries: Promising higher energy density and safety by replacing liquid electrolytes with solid ones.
LFP (Lithium Iron Phosphate) Batteries: These use iron instead of cobalt or nickel, offering lower costs and improved stability.
The production process is capital-intensive and requires precision engineering, making it a critical bottleneck in the value chain.
4. Battery Assembly
Cell manufacturing is followed by assembling cells into modules and battery packs, customized for specific applications such as EVs, portable electronics, or grid-scale storage systems.
Advancements in Assembly:
Thermal Management: Ensuring efficient heat dissipation for safety and longevity.
Pack Design Optimization: Reducing weight and maximizing energy density.
Automakers like Tesla and BYD have pioneered integrated designs that streamline production and improve performance.
5. Deployment and Use
Once batteries are manufactured, they are deployed in various applications:
Electric Vehicles: The EV market is the largest driver of battery demand, expected to grow exponentially.
Energy Storage Systems: Batteries play a critical role in stabilizing renewable energy sources like solar and wind.
Consumer Electronics: Devices such as smartphones, laptops, and wearables rely on batteries for portability.
Challenges in Deployment:
Range Anxiety: Limited battery capacity in EVs can deter consumers.
Charging Infrastructure: The availability of fast-charging stations is crucial for widespread adoption.
6. Recycling and Reuse
As the global demand for batteries surges, so does the need for effective recycling to mitigate environmental impact and reduce resource dependency.
Key Techniques in Recycling:
Pyrometallurgical Processes: High-temperature methods to extract metals from used batteries.
Hydrometallurgical Processes: Using chemicals to recover materials with higher precision.
Direct Recycling: Retaining the structure of components like cathodes for reuse.
Companies like Redwood Materials and Li-Cycle are leading the charge in creating closed-loop recycling systems.
Opportunities in the Battery Value Chain
Sustainable Mining: Technologies such as autonomous mining and low-impact methods can minimize environmental degradation.
Localized Supply Chains: Establishing processing and manufacturing hubs in different regions can enhance resilience.
Battery Second Life: Used EV batteries can be repurposed for less demanding applications, such as stationary energy storage.
Digital Innovations: Blockchain and AI can improve traceability and optimize operations across the value chain.
Challenges in the Battery Value Chain
Despite its potential, the battery value chain faces numerous challenges:
Resource Scarcity: The finite availability of critical materials poses long-term risks.
Geopolitical Dependencies: Over-reliance on specific countries for mining and processing creates vulnerabilities.
High Costs: Advanced technologies and sustainable practices can drive up production costs.
Environmental Impact: While batteries are central to clean energy, their production can contribute to pollution if not managed sustainably.
Addressing these challenges requires global collaboration, regulatory support, and continuous innovation.
The Future of the Battery Value Chain
The battery value chain is poised for transformative growth, driven by innovation and sustainability imperatives. Emerging trends include:
Circular Economy Models: Emphasizing recycling, reuse, and material recovery to reduce waste.
Next-Generation Chemistries: Moving beyond lithium-ion to sodium-ion, zinc-air, and other alternatives.
Regional Diversification: Expanding supply chains to mitigate geopolitical risks.
Green Manufacturing: Incorporating renewable energy and carbon-neutral processes across the chain.
The Role of Policy:
Governments worldwide are incentivizing battery innovation through subsidies, tax credits, and research grants. Policies like the European Union’s Battery Directive and the U.S. Inflation Reduction Act are critical to accelerating progress.
Conclusion
The battery value chain is a vital pillar of the global transition to sustainable energy systems. Its interconnected processes, from mining to recycling, hold immense potential to drive innovation, create jobs, and combat climate change. However, unlocking its full potential requires addressing environmental, ethical, and logistical challenges with a focus on sustainability.
As industries and governments unite to refine this value chain, the future shines bright—powered by cleaner, smarter, and more efficient batteries.
really greate artical , looking for more :)